Follow Professor Angelo Accardo on LinkedIn or learn more about the research he and his team conduct at TU Delft here and the original news report from TU Delft here. A link to the specific paper in our earlier news report can be found here:
Flamourakis, G., Dong, Q., Kromm, D., Teurlings, S., van Haren, J., Allertz, T., Smeenk, H., de Vrij, F. M. S., Tas, R. P., Smith, C. S., Brinks, D., & Accardo, A. (2025). Deciphering the Influence of Effective Shear Modulus on Neuronal Network Directionality and Growth Cones’ Morphology via Laser‐Assisted 3D‐Printed Nanostructured Arrays. Advanced Functional Materials, 35(5), 2025. https://doi.org/10.1002/adfm.202409451
In a groundbreaking study, Professor Angelo Accardo and his team have developed 3D-printed nanopillars that closely mimic the brain’s extracellular environment, offering a novel platform for studying neuron growth, neurodegenerative diseases, and potential applications in personalised medicine.
As part of our in-depth exploration of this research, previously featured in our news coverage, we spoke with Professor Accardo to gain further insights into the technology, its challenges, and its future applications.
The following interview is presented unedited to preserve Professor Accardo’s original responses and provide an in-depth, unfiltered perspective on his work. This format allows readers to fully appreciate the nuances and significance of his research in advancing neuroscience and bioengineering.
Could you explain the specific ways in which the 3D-printed nanopillars mimic the brain’s extracellular environment, and how this benefits neuron growth studies?
The 3D printed nanopillars feature few hundred nanometers diameter, which resemble the one of brain extracellular matrix fibers, as well as the one of filopodia (the “fingers” employed by cells to crawl and probe the surrounding environment). Their aspect ratio can also be tuned to feature a relatively low effective shear modulus (i.e., the shear modulus sensed by cells when crawling on top of the nano-structures’ arrays) that approaches the softness of real brain tissue. These topographic and mechanical cues have thus a profound influence on the growth and directionality of neuronal networks.
What are the key material properties and fabrication techniques used to create these nanopillars, and how do they influence cellular interactions at the nanoscale?
The biomaterial and nanofabrication technique employed in this work are, respectively, a photocrosslinkable polymer, IP-Dip, and two-photon polymerization (2PP). The biomaterial has an intrinsic Young’s modulus (i.e., rigidity) in the GPa range. Nonetheless, by tuning the aspect ratio of the nanopillars, it is possible to achieve effective shear moduli in the few hundreds kPa range. To achieve sub-micrometric feature resolution, we employ 2PP, a fabrication technique based on the use of femto-second near-infrared laser pulses, which trigger photopolymerization (i.e., solidification) of liquid polymer in extremely small volumes, called voxels. By changing the trajectory of the laser beam, it is therefore possible to create any type of 3D free-standing geometry.
How does this platform compare to traditional neuron culture methods in terms of replicating real brain tissue conditions and providing more physiologically relevant insights?
Compared to conventional “petri-dish” approaches, where cells are cultured on flat, plastic or glass, rigid (tens of GPa) surfaces, our approach provide more physiologically relevant topographic and mechanical cues, which greatly influence neuronal cells properties, such as network directionality and morphology of growth cones (by which neurons probe the surrounding extracellular matrix to target other neurons).
Were there any unexpected challenges in designing the nanopillar structures to optimise neuron adhesion, differentiation, and synapse formation?
Two of the main challenges faced in our experiments was the occasional delamination of the nanostructured arrays from the underlying substrate during cell culture, and sample contamination. Since some of our cell culture experiments lasted several weeks, these types of issues could lead to weeks of delay due to the need to restart from scratch the whole culture.
What implications does this research have for studying neurodegenerative diseases or brain injury recovery, and could this technology be adapted for personalised medicine applications?
Neuronal network development and growth cone morphology can be affected by neurodegenerative diseases such as Alzheimer’s (AD) and Parkinson’s disease (PD). With our approach, we demonstrated how is it possible to guide the formation of physiologically relevant neuronal networks and characterize growth cone morphology in a quantitative way. I therefore foresee the use of our platform as an engineered in-vitro disease model to understand the influence of the main hallmarks of AD (amyloidal plaques, neurofibrillary tangles) and PD (Lewy bodies) on neuronal network directionality and growth cone development. The model will therefore represent a bridge between conventional petri-dish approaches and real brain tissue.
How scalable is this 3D printing approach for larger tissue models, and are there potential applications for integrating it with organ-on-a-chip or bioelectronic devices?
Scalability is one the main issues of this fabrication technique nowadays, as the price for sub-micron resolution is high fabrication time. For this reason, we were limited to millimetric areas of nanopillars. There is a lot of development though in the 2PP field to increase fabrication speed, by using two-photon gray scale lithography, adaptive resolution, new optics, galvo mirrors and acousto-optic deflectors, or multi-beam 2PP systems to name a few. On the other hand, the integration of these nanostructured devices within organ-on-chip is already a reality and we obtained very promising results that we hope to publish this year.
Looking ahead, what are the next steps for your research? Are there plans for collaborations with neuroscience or pharmaceutical teams to further develop the technology for clinical or drug discovery applications?
At the moment, I have two ongoing NWO projects on the influence of environmental pollution on 3D engineered Alzheimer’s models, and on 3D cell microenvironments to study genetic disorders associated with autism spectrum disorder. In future, I plan to interact with pharma to assess the efficiency of our models as in-vitro drug screening platforms. In another project on 3D engineered brain cancer microenvironments, in collaboration with the Holland Proton Therapy Center, we recently developed a benchmark tool for proton radiobiology that we plan to further develop in future to assess the efficiency of radiation treatments on glioblastoma, the deadliest and most aggressive brain cancer.
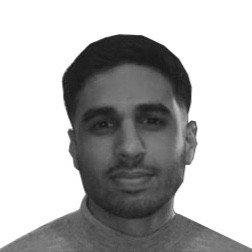
Hassan graduated with a Master’s degree in Chemical Engineering from the University of Chester (UK). He currently works as a design engineering consultant for one of the largest engineering firms in the world along with being an associate member of the Institute of Chemical Engineers (IChemE).