In a groundbreaking advance Dr. Maksud Rahman and his group at The University of Houston have demonstrated a stereolithography-based route to origami-inspired ceramic structures that marry high-resolution printability with foldable flexibility. Featured in our previous news article, the team utilised a silica-loaded photopolymer resin and a hyperelastic coating strategy, their work can be used in multiple industries such as aerospace components, armour, and beyond. As part of our in-depth coverage of this emerging field, we spoke with Dr. Rahman to explore the formulation, 3D-printing and sintering processes, mechanical performance, and scale-up challenges of these bendable ceramics.
Follow the authors here:
- Md Shajedul Hoque Thakur | Linkedin
- Methu Dev Nath
- Pulickel M. Ajayan
- Glaucio H. Paulino | Linkedin
- Dr. Maksud Rahman | Linkedin
The main research paper pertaining to this interview can be found below:
Thakur, M. S. H., Nath, M. D., Ajayan, P. M., Paulino, G. H., & Rahman, M. M. (2025). Macroscale ceramic origami structures with hyper-elastic coating. Advanced Composites and Hybrid Materials, 8(2), 226. https://doi.org/10.1007/s42114-025-01284-3
The following interview is presented unedited to preserve Dr. Rahman’s original responses and provide an unfiltered look at his pioneering work in ceramic origami design.
What ceramic formulations and particle sizes are you using to achieve both printability and the flexibility required for origami folding, and how did you optimise that balance?
The formulation we are using is the Formlabs Ceramic Resin V1, it is their proprietary formulation. It is a photopolymer resin with silica particles dispersed within it. The size of the silica particles is ~5m. The photopolymer enables printability via stereolithography as it solidifies upon exposure to light. The flexibility doesn’t come from the formulation, rather it comes from the origami design and the hyperelastic coating that is later applied to the printed and sintered ceramic origami structure.
Can you walk us through the 3D-printing process; layer orientation, binder chemistry, sintering profile, that enables the printed ceramic to exhibit hinge-like behaviour without cracking?
The 3D-printing process used is a stereolithography technique. It prints by shining a laser into a tank of liquid resin, hardening it layer by layer to create the object. The structures are printed at an arbitrary orientation, distinct from the three orthogonal orientations in which mechanical properties are measured, as shown in the image below –
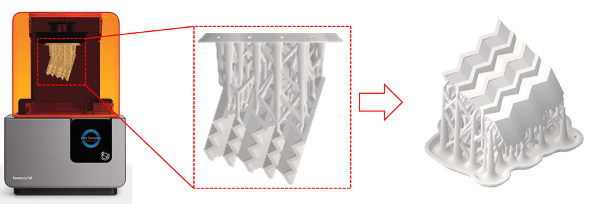
The printed structure are heated in accordance with the following firing schedule –
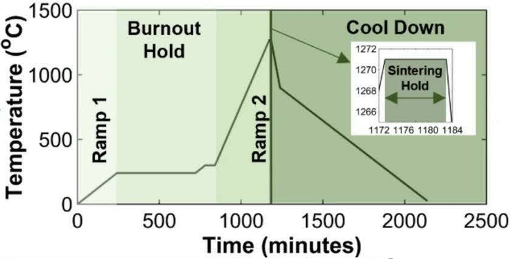
The first burnout hold at 240 °C removes the photopolymer. At this stage, the silica particles have not yet fused and are loosely held together as a powder body. This phase should be long enough to completely remove the photopolymer. Partial burnout will cause vapor pressure to increase during the ramp phase, resulting in cracks and distortion as vapor escapes. A short secondary hold at 300 °C ensures that all polymer is burned out before Ramp 2. Ramp 2 heats up the part to the sintering temperature of 1271 °C at a ramp rate of ~ 3 °C per minute. Finally, to ensure fully sintered ceramic parts with minimal crack formation we cool down at 1 °C per minute, and finally at 0.5 °C per minute.
How do the geometric design parameters (e.g., fold angle, crease thickness, unit cell topology) influence the mechanical performance and elastic recovery of the origami structures?
This particular Miura-ori design gives high anisotropy to the structure. The structure is extremely stiff and tough in one direction (Z) and quite compliant in comparison in the X direction, while the Y direction has moderate stiffness. Further research is needed to pinpoint the effect of each individual variable such as the fold angles and crease thickness.
What mechanical testing have you performed (fatigue, bending radius, load-displacement) to quantify durability over repeated folding cycles, and what failure modes have you observed?
The X-direction is the most compliant orientation, we carry out cyclic compression tests on the coated origami structure in the X-direction. The cyclic loading is done up to 1.5% compressive strain because the uncoated samples consistently fail before ~1.5% compressive strain. We cycled it 10 times to this strain and it did not fail except a small crack at one compartment, while the uncoated fails catastrophically at this strain.
How does the high-temperature stability and thermal shock resistance of these bendable ceramics compare to conventionally processed monolithic ceramics?
We have not yet looked into that, but that’s a possible research direction.
What are the key challenges in scaling this technique; both in terms of print volume and complexity of folding patterns, and how might you overcome limitations like warpage or binder burnout?
The key challenge in scaling it up to larger origami structures is the viscosity of the ceramic resin. The Formlabs ceramic resin is an experimental resin, and due to the silica particles dispersed in it, its viscosity is very high. This makes it challenging to print complex architectures such as origami with this resin, particularly in large volumes as that requires too many supports are difficult to remove without compromising the structure. So the key challenge in scaling this up lies in modifying the ceramic resin or using the new printing process, which are all possible future research directions. This study is a fundamental aspect of solving an engineering problem.
Looking toward applications, which fields (e.g. deployable aerospace structures, high-temperature sensors, compliant armour) do you see as most promising, and what further material or process developments are needed to move toward commercialisation?
We see this as most applicable for prosthetics as well as compliant armour. The developments needed to move toward commercialisation need to be evaluated further through the modifying the ceramic resin or using the new printing process.
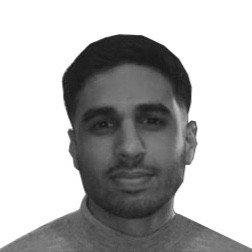
Hassan graduated with a Master’s degree in Chemical Engineering from the University of Chester (UK). He currently works as a design engineering consultant for one of the largest engineering firms in the world along with being an associate member of the Institute of Chemical Engineers (IChemE).