Although the water-splitting process has been a leading idea for creating hydrogen fuel in a sustainable and eco friendly way for years, researchers have always pointed out that consistently, the real-world energy input required exceeds theoretical calculations. Results at Northwestern University, which have been published in Nature Communications, paint a more detailed picture about where these differences in energy are coming from and propose ways for improvement. We featured their previous work which you can view here. You can also view the published paper here:
Speelman, R., Marker, E. J., Boamah, M. D., Kupferberg, J., Bye, J. Z., Engelhard, M., Zhao, Y., Martinson, A. B. F., Rosso, K. M., & Geiger, F. M. (2025). Water flipping and the oxygen evolution reaction on Fe2O3 nanolayers. Nature Communications, 16(1), 3585. https://doi.org/10.1038/s41467-025-58842-y
Water splitting involves applying an electrical voltage to water, forcing it to separate into hydrogen and oxygen. Ideally, the two half-reactions involved; one for hydrogen production and one for oxygen production, would occur at a predictable, affordable energy cost. In practice, however, the oxygen production requires an extra voltage, which has been measured at between 1.5 and 1.6 volts, well above the expected 1.23 volts. Franz Geiger, a Charles E. and Emma H. Morrison Professor of Chemistry at Northwestern’s Weinberg College of Arts and Sciences and member of the International Institute for Nanotechnology, who led the study said:
“When you split water, two half-reactions occur, one half-reaction produces hydrogen and the other produces oxygen. The half-reaction that produces oxygen is really difficult to perform because everything has to be aligned just right. It ends up taking more energy than theoretically calculated. If you do the math, it should require 1.23 volts. But, in reality, it requires more like 1.5 or 1.6 volts. Providing that extra voltage costs money, and that’s why water splitting hasn’t been implemented at a large scale. We argue that the energy required to flip the water is a significant contributor to needing this extra energy. By designing new catalysts that make water flipping easier, we could make water splitting more practical and cost-effective.”
Franz Geiger was joined by, the study’s lead author Raiden Speelman and second author Ezra Marker, who are both members of Geiger’s lab. Other co-authors include Alex Martinson from Argonne National Laboratory and Mavis Boamah, Jacob Kupferberg, Mark Engelhard, Yatong Zhao and Kevin Rosso; all from the Pacific Northwest National Laboratory.
The study used a sophisticated optical method known as phase-resolved second harmonic generation (PR-SHG). Using this technique, the researchers observed how water molecules interact with a hematite electrode; a common, cost-effective iron oxide material. The experimental setup involved applying a controlled voltage while monitoring the alignment of water molecules using laser-based measurements.
The findings indicate that when water molecules initially approach the electrode, they adopt a random orientation. As the voltage increases, an electric field forces the water molecules to flip; the hydrogen atoms move away from the electrode so that the oxygen atoms can facilitate electron transfer. This reorientation, while necessary for the oxygen evolution reaction to proceed, also imposes a significant energy cost. The study further notes that the energy needed for this flipping closely aligns with the energy holding liquid water together.
Earlier work by Geiger’s team on nickel electrodes showed a similar reorientation process, suggesting that the flipping mechanism is not unique to one type of electrode material.
Another important factor is the influence of the water’s pH on efficiency. Research indicates that at higher pH levels, the energy required to achieve the proper molecular alignment drops. On the other hand, lower pH conditions demand considerably more energy, effectively throttling the current produced during the reaction.
The search for cost-effective and efficient electrode materials is continuously evolving. While iridium has shown some success due to its favourable catalytic properties, its scarcity and cost are major drawbacks. The used of more readily available and affordable materials like hematite and nickel, is a more sustainable and economical approach towards hydrogen fuel production at a larger scale. Geiger said:
“Iridium only comes to Earth from meteoric impact, like the famous iridium anomaly at the Cretaceous-Paleogene boundary, so there’s a limited amount, It’s very expensive and certainly not going to help solve the energy crisis any time soon. Researchers are looking at alternatives, like nickel and iron, and we’re hoping to find ways to make these materials just as efficient; if not more efficient, than iridium.”
Studies like the one by Northwestern researchers show that the catalyst surfaces need to be tailored to facilitate water flipping so the electron transfer can be initiated. The goal with research like this is to move away from fossil fuels and toward a hydrogen economy.
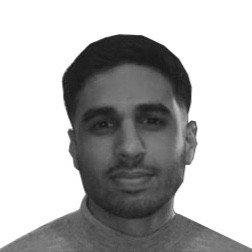
Hassan graduated with a Master’s degree in Chemical Engineering from the University of Chester (UK). He currently works as a design engineering consultant for one of the largest engineering firms in the world along with being an associate member of the Institute of Chemical Engineers (IChemE).