Each reactor type utilises mechanisms for cooling and moderation, directly impacting their operational efficiency, fuel usage, and safety measures. For example, AGRs are known for achieving high thermal efficiencies through high-temperature operations. The simplicity and compactness of BWRs that boil water directly in the reactor core is also a common attribute associated with BWRs. Most details about these particular reactors will be explored along with their operational benefits and their contemporary use in the energy industry.
To learn more about Nuclear Reactors or just to get a good headstart on your education or to bolster your professional career, check out the following book which has been recommended by numerous industry professional and professors alike:
Different Types of Advanced Nuclear Reactors:
- Advanced Gas-cooled Reactors (AGRs),
- Pressurized Water Reactors (PWRs),
- Boiling Water Reactors (BWRs),
- Pressurised Heavy Water Reactors (PHWRs).
What Are Advanced Gas-cooled Reactors (AGRs) and How Do They Work?
AGRs make use of carbon dioxide as a coolant and graphite as a moderator, thereby allowing them to operate at high temperatures, hence greater thermal efficiency, usually around 40%. This is because, unlike water, carbon dioxide is stable at high temperatures without causing phase change (e.g., boiling) under the conditions designed in AGRs. High operating temperatures allow a higher percentage of the heat generated by fission to be converted into mechanical and thus electrical energy therefore, higher thermal efficiency.
Graphite is an effective neutron moderator even at high temperature. Its characteristic moderation of the fast neutrons without a significant loss of moderating efficiency at high temperature makes it a highly suitable material for a reactor core where high-temperature operation is required such as AGRs.
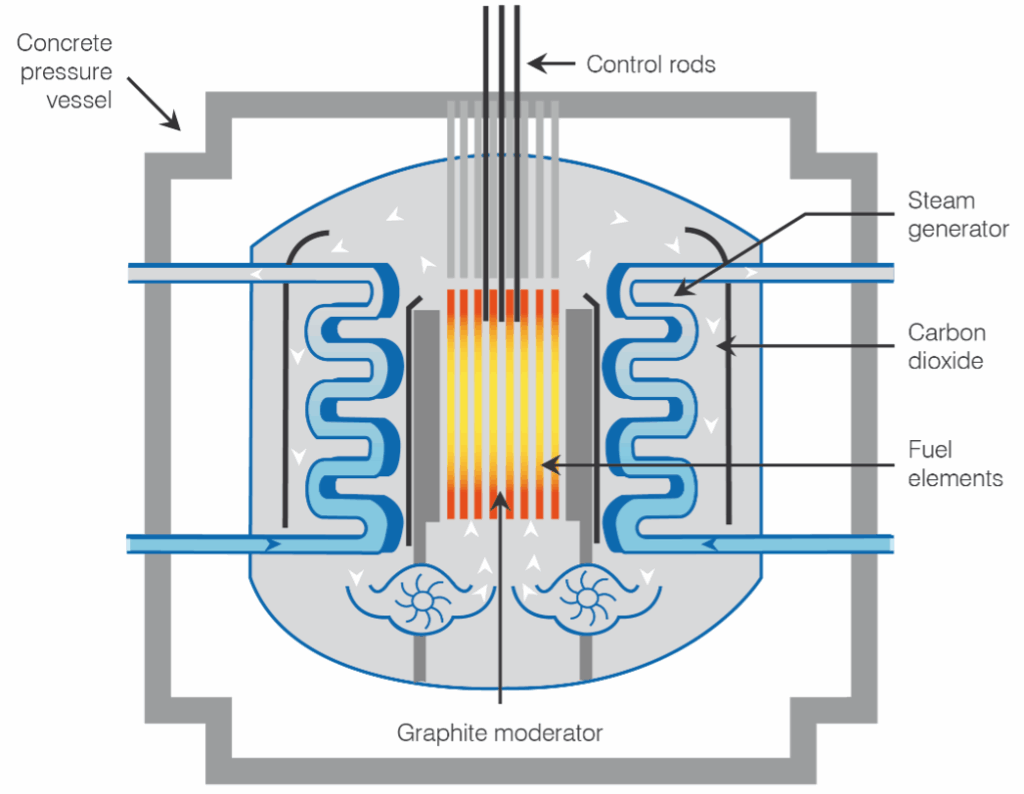
This choice of coolant and moderator also allows a wider range of uranium fuels to be used. However the construction and maintenance of the said reactors is far more expensive compared to their counterparts due to the materials required for high-temperature operations.
What Are Pressurized Water Reactors (PWRs) And How Do They Work?
Pressurized Water Reactors (PWRs) are one of the most widespread and most used types of nuclear reactors. They utilise water for two primary purposes: cooling the core of the reactor and moderating (slowing down) neutrons emitted during fission.
Within the reactor core, the uranium fuel undergoes nuclear fission, where the uranium atoms disintegrate and release heat. Heat is generated as fast neutrons are slowed down through collision in the water, which acts as a moderator. The moderated neutrons are more probable to trigger subsequent fission reactions, sustaining the chain reaction in a controlled manner.
The water circulated through the core serves a dual purpose. It removes the heat produced by fission, acting as a coolant, and slows down neutrons acting as a moderator. The pressuriser maintains pressure on the water. The high pressure, increases the boiling point of water, thus, preventing the water from boiling even at such temperatures as it reaches, allowing it to efficiently carry heat away from the reactor core.
After absorbing heat in the reactor core, the pressurised heated water travels through a heat exchanger known as the steam generator. In the steam generator, the heat from the primary water circuit is transferred to a separate water system. This secondary water is not under high pressure and is allowed to boil, creating steam.
The steam produced in the secondary loop is directed to turbines. As the steam expands and cools, it drives the turbine blades, which in turn rotates a generator. This mechanical energy conversion produces electricity. After passing through the turbine, the steam is usually condensed back into water and recirculated to be heated again, completing the cycle.
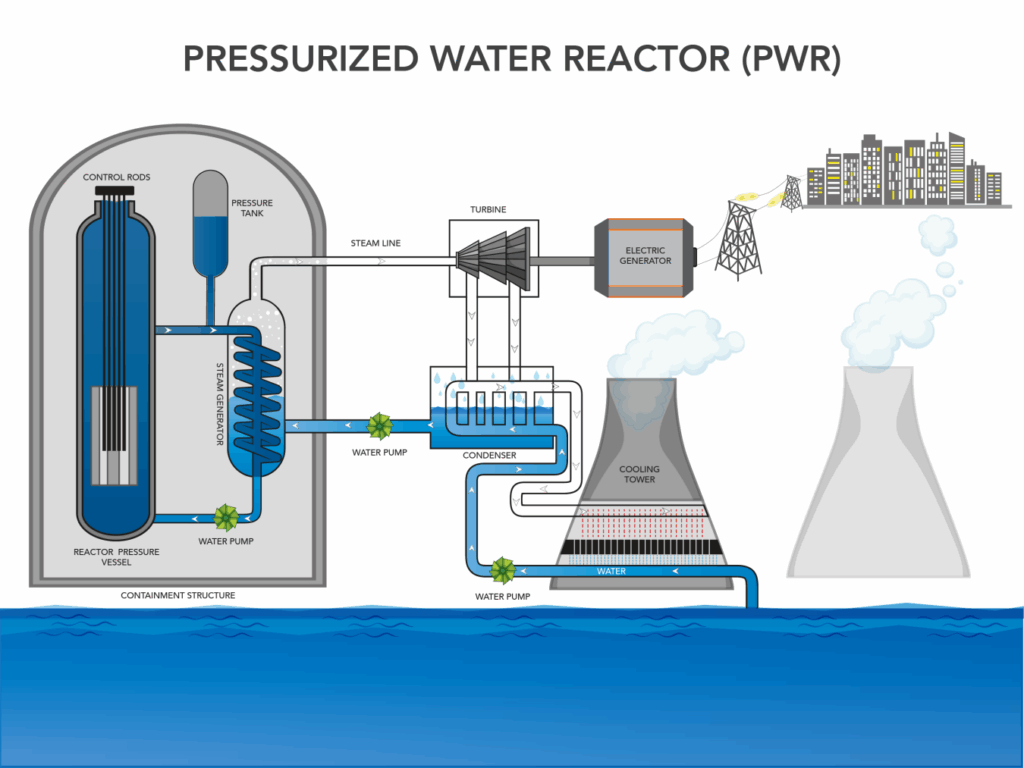
Overall, the design of a PWR; with its high-pressure primary water loop and a separate secondary steam cycle, provides a reliable and efficient means of harnessing nuclear energy while ensuring safety and operational stability.
What Is A Boiling Water Reactor (BWR) and How Does It Work?
A Boiling Water Reactor (BWR) works by placing uranium fuel assemblies within the reactor core, where nuclear fission generates both heat and fast neutrons. When these neutrons strike water molecules that are being circulated through the core, the water heats up and, at the same time, serves as a moderator, cooling the neutrons down enough to allow for a controlled chain reaction. Because the coolant water is allowed to boil when it comes into contact with the fuel, steam is produced directly in the reactor vessel; eliminating the need for a separate steam generator loop like in the PWRs.
Once produced, the steam flows directly from the reactor core to the turbine, causing its blades to spin and driving an electric generator. Upon exiting the turbine, the steam is channeled into a condenser, where it is cooled, condensed back to liquid, and pumped back into the reactor vessel to loop again. Internal recirculation variable speed motor pumps inside the reactor use the water flow rate through the core so that the boiling rate and hence the reactor’s power level can be adjusted by the operators without needing to shift control rods.
Control rods, made out of neutron-absorbing elements. Common types of material used to make the control rods are: Boron Carbide (B₄C) and Hafnium. The control rods are added from above to regulate the fission rate and provide for safe levels of reactivity. On a sudden decrease in pressure or water levels, a number of emergency core cooling systems can flood additional coolant into the system in order to prevent overheating. All this within a sturdy containment building designed to protect against the release of any radioactive material upon an accident occurring.
BWRs are relatively compact, since fewer mechanical and heat‑exchange loop elements are required. However, because the steam employed to drive the turbine is radioactive, rigorous water chemistry controls must be implemented to minimise corrosion and limit the formation of radioactive deposits. BWRs typically have thermal efficiencies of around 32–34%, with operating pressures of around 7 MPa and temperatures of around 285 °C. This combination of simple steam production and minimised circuitry makes BWRs cost-effective to build and simple to operate but still demanding fine control of materials and safety protocols.
How Is a BWR Different From a PWR Reactor?
Compared to the secondary loop PWRs, the steam that is generated in the BWR core is utilised to directly drive a turbine that is connected with a generator to produce electricity. The steam is thereafter condensed into water in a condenser and recycled back to the reactor core for reheating. Control rods, made of neutron-absorbing materials, are inserted into or withdrawn from the reactor core to control the rate of fission and, consequently, the quantity of steam produced. This direct cycle of heating water to produce steam in the reactor simplifies the design and operation of BWRs, without a separate steam generator and with a less cumbersome system.
Safety systems are in place to cool the reactor and contain radioactive materials in the event of an emergency, ensuring the safe operation of the reactor.
What Are PHWRs And How Do They Work ?
Pressurised Heavy Water Reactors (PHWRs) are characterised by their use of heavy water (D2O) for both cooling and moderating neutrons, enabling the efficient use of natural uranium as fuel. Heavy water has a much lower neutron‑absorption cross‑section. This means that when fast neutrons from fission collide with D₂O molecules, they are slowed (moderated) into the thermal energy range without being captured as readily as they would be in H₂O. Although heavy water behaves chemically like ordinary water, each molecule contains deuterium atoms (hydrogen isotopes with an extra neutron), making it about 11% denser.
This design allows for significant moderation of neutrons, increasing the probability of nuclear fission reactions without necessitating the enrichment of uranium. The heavy water circulates around the reactor core, collecting the heat caused by fission, and transfers it to a secondary water circuit via a heat exchanger, creating steam without direct contact between both circuits. The steam powers turbines that produce electricity, and the steam is afterwards condensed and recycled.
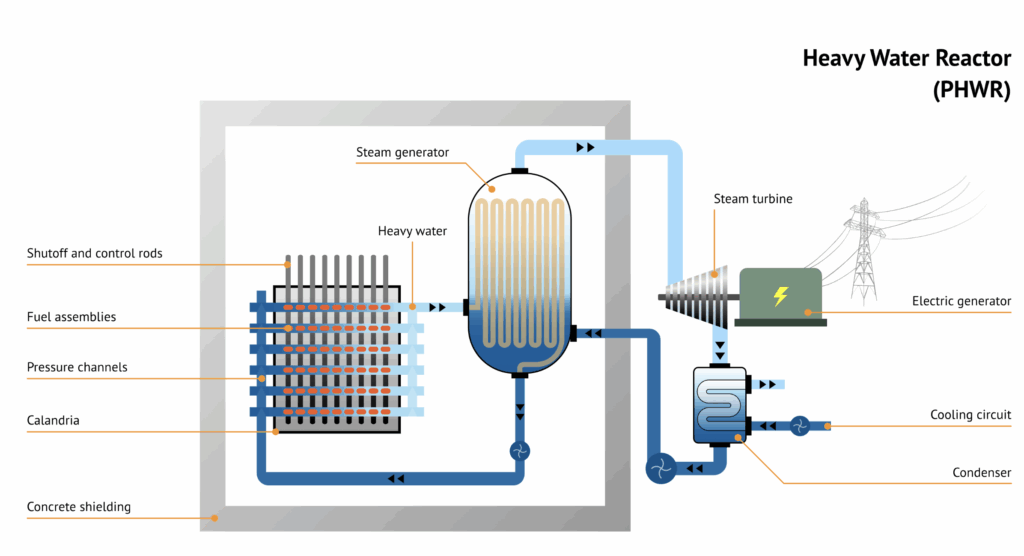
PHWRs are fueled with natural uranium dioxide pellets, which are contained in rods that are clustered together, and utilise control rods to regulate the fission rate and power. One of the distinctive advantages of PHWRs, particularly of the CANDU design, is that they have the capability for online refueling, i.e., they can be operated continuously without being shut down to replace the fuel. This operational flexibility, together with the possibility of using unenriched uranium, is what PHWRs provide economically despite the added cost and complexity of heavy water use.
Advantages and Disadvantages of Different Types of Nuclear Reactors
Reactor Type | Advantages | Disadvantages |
---|---|---|
Advanced Gas-cooled Reactors (AGRs) | High thermal efficiency (~40%) due to stable CO₂ coolant at High temperatures Graphite moderator retains good performance at high temperatures Fuel flexibility (can use a variety of uranium fuels) | High capital and maintenance costs from specialised high‑temperature materials Complex CO₂ coolant system requiring precise engineering Graphite core ageing and inspection challenges |
Pressurized Water Reactors (PWRs) | Low Learning Factor, widely deployed design with extensive operational experience Separate primary/secondary loops minimise radioactive contamination in turbines Robust safety systems and proven pressuriser technology | Moderate thermal efficiency (≈33%) compared to high‐temperature designs Very high operating pressure necessitates thick, expensive reactor vessel Pressuriser adds complexity and potential failure modes |
Boiling Water Reactors (BWRs) | Simplified, compact design (direct steam generation in reactor core) Fewer heat‑exchange components reduce capital cost Lower operating pressure than PWRs | Radioactive steam directly drives turbines, requiring more shielding and maintenance Slightly lower thermal efficiency (≈32%) More stringent water chemistry control to prevent corrosion |
Pressurized Heavy Water Reactors (PHWRs) | Can run on natural (unenriched) uranium, lowering fuel costs Online refueling enables continuous operation and high capacity factor Heavy water moderator provides excellent neutron economy | High cost of heavy water production and inventory Heavy‑water leak detection and recovery systems add complexity Thermal efficiency is moderate (≈30%) compared to high‑temperature designs |
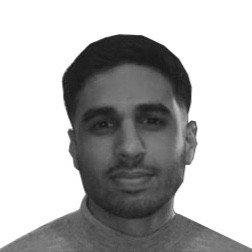
Hassan graduated with a Master’s degree in Chemical Engineering from the University of Chester (UK). He currently works as a design engineering consultant for one of the largest engineering firms in the world along with being an associate member of the Institute of Chemical Engineers (IChemE).