Scientific experts have been perplexed by how platinum electrodes degrade during negative polarisation for many decades. Platinum demonstrates superior stability and durability making it essential for electrolysers and electrochemical devices used in green hydrogen production. Platinum exhibits rapid corrosion while maintaining negative polarity within electrolytes that include saltwater solutions.
Researchers from Leiden University, in collaboration with the Department of Energy’s SLAC National Laboratory, have now identified the primary cause; hydride formation on the platinum surface. The researchers published their work in Nature Materials while providing guidelines for producing durable electrodes that will advance sustainable green technology development.
Under typical conditions, metals resist corrosion when negatively polarised. However, platinum behaves differently. When exposed to a highly negative potential in an electrolyte (Saltwater), platinum can degrade in minutes, a significant issue for technologies like electrolysers, which rely on its stability.
“Being quite stable doesn’t mean it doesn’t degrade at all,”
says Dimosthenis Sokaras, a senior scientist at SLAC and the SLAC team’s principal investigator.
“If you take a piece of platinum and you apply a very negative potential, you can dissolve your platinum in a matter of minutes,”
Stated Marc Koper, Professor of Catalysis and surface chemistry at Leiden University, and the Leiden team’s principal investigator.
To uncover the mechanism behind platinum’s corrosion, the researchers employed cutting-edge tools at SLAC’s Stanford Synchrotron Radiation Lightsource (SSRL). High-energy-resolution X-ray absorption spectroscopy, a technique capable of examining subtle atomic changes during operation, was pivotal. This approach allowed the team to study platinum electrodes in real time, even as hydrogen bubbles; common in such experiments, were cleared using a specialised flow cell.
“High-energy-resolution X-ray absorption spectroscopy, for us, was the only technique we could come up with that could sort of deal with the experimental conditions,”
stated SLAC scientist Thom Hersbach.
By combining spectroscopy with advanced computational models, the team simulated the X-ray spectra expected from both platinides and hydrides. Their analysis confirmed that hydrides, not platinides, were responsible for the corrosion. These findings marked the first direct observation of hydride-driven degradation in platinum electrodes.
This discovery provides critical insights for addressing platinum’s limitations in electrochemical systems. Scientists and engineers can now work on designing more robust platinum-based materials or alternative solutions to minimise hydride formation. This could lower the costs of green hydrogen production and enhance the longevity of devices like sensors and fuel cells.
“This project shows how important in science it is to bring together expertise from different fields.”
Sokaras stated. By bridging experimental physics, computational modelling, and materials science, the team tackled a problem that had eluded definitive answers for years.
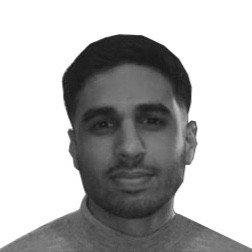
Hassan graduated with a Master’s degree in Chemical Engineering from the University of Chester (UK). He currently works as a design engineering consultant for one of the largest engineering firms in the world along with being an associate member of the Institute of Chemical Engineers (IChemE).