Follow Dr. Imogen Riddell on LinkedIn or learn more about the research she and her team conduct at the University of Manchester here. A link to the specific paper related to our earlier news report and this interview can be found here:
Wright, J. D., Whitehead, G. F. S., Pyzer-Knapp, E. O., & Riddell, I. A. (2025). Encapsulation of hydrophobic pollutants within a large water-soluble [Fe4L6]4− cage. Cell Reports Physical Science, 102404. https://doi.org/10.1016/j.xcrp.2025.102404
In a groundbreaking study, Dr. Imogen Riddell and her team have developed a molecular trap technology designed to selectively capture pollutants from water, addressing a critical challenge in environmental remediation. As part of our in-depth exploration of this topic, which we previously covered in a news feature, we spoke with Dr. Riddell to gain further insight into her research and its potential impact on water purification.
The following interview is presented unedited to preserve Dr. Riddell’s original responses, offering readers an unfiltered look at the science behind this innovative approach and its implications for sustainability and environmental protection.
Acknowledgement From Dr. Imogen Riddell: This work was supported by a The Royal Society (UK) University Research Fellowship (IAR; URF∖R1∖180414), including a contribution to a PhD studentship (J.D.W.). We also thank the EPSRC and NMR infrastructure (EP/K039547/1 and EP/R00482X/1) and the Diamond Light Source for access to synchrotron X-ray facilities at Beamline I-19 (cy31541). I.A.R. and J.D.W. also thank Prof. Simon Webb for helpful discussions and gift of ethinylestradiol.
Could you explain the fundamental mechanism behind the molecular trap technology and how it selectively captures pollutants from water?
When designed correctly, molecular traps, or metal-organic cages (MOCs), readily self-assemble from metal ions and organic ligands. Ideally a single type of MOC is generated during this self-assembly process, which has a well-defined binding pocket. This MOC can then be used to selectively capture chemicals, such as the environmental pollutants we specifically targeted here.
Once assembled in aqueous solution the MOC is available for guest / pollutant binding. Our MOC takes advantage of the hydrophobic effect. Effectively what is happening upon binding in our cage is that the water repelling (hydrophobic) pollutants are preferentially located inside of our MOC, where they are shielded from the water molecules. At the same time, water molecules prefer to be outside the internal binding space than trapped inside it and they are therefore readily displaced by the incoming pollutants.
In order to achieve the MOCs in this study, we also had to overcome an additional challenge, as there is a synthetic challenge to building MOCs with the desired binding pockets that are also soluble in water. This is because there is a fundamental synthetic chemical challenge to creating ligands that have both the required hydrophobic/ water repelling properties and good water-solubility. These mismatched properties are however necessary if you are to access structurally rigid MOCs with hydrophobic binding pockets that are water-soluble.
What types of pollutants does this material target most effectively, and how does it compare to existing filtration or absorption methods in terms of efficiency and selectivity?
Our studies focused on established environmental pollutants found at low concentrations in wastewater discharge. Wastewater processing facilities vary country to country, with discrepancies in both plant capacity and purification technologies. It is clear however from environmental studies that across the western world the chemicals we have targeted are causing substantial damage to our ecosystems thus new approaches are required to reduce the concentration of these chemicals expelled into our nature water systems.
Our MOC specifically targets hydrophobic molecules in aqueous wastewater. It is therefore selective for molecules that are of an appropriate size and hydrophobicity to fit within the central binding pocket. This shape and property selectivity differs from a filtration method which is only discriminatory with respect to size.
For example, we find that griseofulvin, an antifungal medicine, had insufficient hydrophobicity and fluticasone propionate, an anti-inflammatory drug, was too large for the binding pocket, and therefore neither bound within the MOC. Future work will look at development of related cages with suitable properties for capture of pollutants with a different spectrum of physical and chemical properties.
In terms of efficiency, each MOC has sufficient internal volume to capture one pollutant molecule and can do so on short timescales with binding affinities up to 280,000 M-1. Therefore, coupling this chemical separation with a robust, engineered recycling procedure, has the potential for high level water purification.
What are the key structural or chemical properties of this material that enable its high absorption capacity, and how does it maintain stability in real-world water treatment conditions?
There are two key features of our MOC which enables binding of hydrophobic pollutants in water: i) it is water-soluble with a hydrophobic cavity so there is a driving force to bind matched molecules and ii) the cavity is sufficiently large that it can accommodate our targeted pollutants. Specifically, the aromatic panels at the centre of each ligand form an attractive binding pocket at the centre of the MOC enabling binding of conjugated, polycyclic guests such as tonalide and ethinylestradiol. At the same time the dimensions of these pockets limit access to larger, bulkier molecules providing a size selectivity filter.
Data collected to date indicates that our MOC has good stability in water over a period of 1 month but extensive testing is required to understand stability at longer timeframes and in the presence of the broad range of established waste water components, this includes both man-made contaminants and those arising from natural processes such as degradation of vegetation etc.
How scalable is the synthesis of this molecular trap for industrial water purification applications, and what are the main challenges in large-scale production?
A bottleneck to many MOC preparations is the synthesis and purification of the organic ligands. Our ligand synthesis procedure is high yielding and the procedure has precedent in industrial processes. Purification of the ligand is by crystallization which is simple to scale up and importantly our purification approach doesn’t rely on time consuming, poorly scaling techniques such as chromatography. Similarly, the MOC synthesis utilises a self-assembly process which is inherently scalable and can be purified by precipitation or crystallization both of which scale well.
Does the material allow for pollutant recovery or regeneration for reuse, and how does this impact the sustainability and long-term viability of the technology?
Preliminary results have indicated release of the pollutant and recycling of the MOC is possible however this is an ongoing area of research for our lab. In terms of the stability of the MOC we see no degradation over several weeks in pure water, but further work is required to better understand the role of possible contaminants and extreme environmental conditions.
An established route to recycling with similar types of materials is to immobilise the trap on a surface or in a membrane, capture the desired contaminant and then empty/ regenerate the trap by flowing an alternative solvent over the immobilised trap. We are actively pursuing whether this is possible through modification of our system as obviously this would make a significant impact with regard to sustainability.
What are the potential applications beyond water purification? Could this technology be adapted for air filtration, chemical sensing, or other environmental remediation efforts?
MOCs are established for capture of contaminants, greenhouse gases and separation of chemicals. The innovation here is that we have generate a water-soluble, rather than organic soluble, MOC, which also has a large cavity. This allows our MOC to be applied to different problems, rather than for example fine chemical purification we can look at chemical sequestration from water.
At a fundamental level our MOC is able to operate in aqueous solutions and could be adapted for application to any relevant problem where the chemical of interest is hydrophobic and of appropriate size. This includes application for example in drug delivery or use in development of green catalysts.
Looking forward, what are the next research steps for improving or expanding the capabilities of this molecular trap, and are there any ongoing collaborations with industry or government bodies to accelerate its real-world implementation?
There are lots of exciting avenues for future work with these systems including the development of new cages, robust recycling protocols and the application of cages to areas as yet unexplored.
Building on the synthetic techniques developed here we are interested to further increase the size and diversity of the internal cavities inside of these MOCs to develop even larger water-soluble cages. Larger MOCs will be able to capture larger molecules and further extend the potential application of these systems. We also want to probe the fundamental design rules of these MOCs, which would allow us to design new MOCs with specific functions, maximising their utility and potentially opening up new application areas for this exciting class of molecule.
Currently there are no ongoing collaborations with industrial or government bodies but it is certainly something we are looking to explore as we further mature this exciting technology.
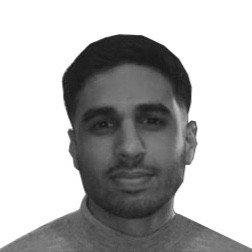
Hassan graduated with a Master’s degree in Chemical Engineering from the University of Chester (UK). He currently works as a design engineering consultant for one of the largest engineering firms in the world along with being an associate member of the Institute of Chemical Engineers (IChemE).