Graphene’s combination of electrical conductivity and extreme tensile strength has made it a sort of wonder material in recent years. Yet its very stiffness; stemming from a honeycomb lattice of carbon atoms, limits applications that demand flexibility or stretchability. Researchers at the University of Vienna have engineered a corrugated, accordion-like structure into graphene sheets, altering their mechanical response and reducing the force required to stretch the material. The research was published in the journal, Physical Review Letters and can be found here:
Joudi, W., Windisch, R. S., Trentino, A., Propst, D., Madsen, J., Susi, T., Mangler, C., Mustonen, K., Libisch, F., & Kotakoski, J. (2025). Corrugation-Dominated Mechanical Softening of Defect-Engineered Graphene. Physical Review Letters, 134(16), 166102. https://doi.org/10.1103/PhysRevLett.134.166102
In a collaboration led by Professor Jani Kotakoski at the Faculty of Physics, University of Vienna, the team introduced vacancies; missing carbon atoms, into graphene membranes via low-energy argon ion irradiation. By controlling the vacancy density to about 1 × 10^13 defects per cm², they induced nanoscale bulges that coalesce into periodic ripples. When tension is applied, these ripples unfold rather than directly stretching the lattice, much like an accordion expanding under pull.
All experiments were performed within an interconnected ultra-high vacuum system. Samples moved seamlessly between a Bruker Nion Ultra STEM 100 scanning transmission electron microscope and a Quantum Design AFSEM atomic force microscope without ever contacting ambient air. This environment prevented adsorption of airborne contaminants; which previous studies had shown can obscure or even reverse softening effects, and ensured that measured changes arose from engineered defects only.
High-resolution STEM imaging confirmed the vacancy distributions, while AFM nanoindentation quantified mechanical properties on the same graphene membrane before and after irradiation. Pristine graphene exhibited a two-dimensional elastic modulus of approximately 286 N/m. After introducing clustered vacancies, the modulus dropped to near 158 N/m, a reduction far greater than most theoretical models had predicted. This pronounced softening is influenced not by bond weakening around individual defects, but by the flattening of corrugations under load. Jani Kotakoski explained:
“This unique system we have developed in the University of Vienna allows us to examine 2D materials without interference,”
Wael Joudi, first author of the study, adds:
“For the first time, this kind of experiment has been carried out with the graphene fully isolated from ambient air and the foreign particles it contains. Without this separation, these particles would quickly settle on the surface, affecting the experiment procedure and measurements.”
To validate the accordion mechanism, theoretical physicists Rika Saskia Windisch and Florian Libisch from the Vienna University of Technology performed atomistic simulations. Their models demonstrated that only vacancies occurring in pairs or larger clusters generate sufficient local strain to create bulges. Single-atom defects produced minimal out-of-plane distortion and negligible impact on stiffness. Under applied tension, simulated rippled graphene showed a marked decrease in stress compared to a flat lattice.
The team also investigated the effect of surface contamination. Introducing trace amounts of adsorbates onto pristine graphene suppressed ripple formation and even appeared to stiffen the material. This finding reconciles conflicting reports in the literature, where variations in sample handling led to inconsistent stiffness measurements. Maintaining an atomically clean surface proved essential for reproducible engineering of mechanical properties.
The work was led by Professor Jani Kotakoski at the University of Vienna, with experimental measurements by first author Wael Joudi; theoretical simulations by Rika Saskia Windisch and Florian Libisch at the Vienna University of Technology. Experiments used a Bruker Nion Ultra STEM 100 microscope from Bruker Corporation and an AFSEM system from Quantum Design GmbH.
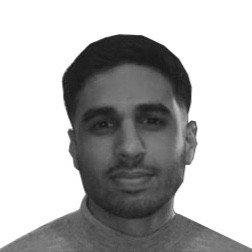
Hassan graduated with a Master’s degree in Chemical Engineering from the University of Chester (UK). He currently works as a design engineering consultant for one of the largest engineering firms in the world along with being an associate member of the Institute of Chemical Engineers (IChemE).