Water, while its crystalline ice phases have been extensively studied, a recent breakthrough at the University of Utah has expanded our understanding of its amorphous forms. Researchers from the Molinero Research Group have used computer simulations to investigate the effects of shear on medium-density amorphous (MDA) ice, giving us fresh ideas of how water’s phase behaviour acts under extreme conditions. See the research here:
de Almeida Ribeiro, I., Dhabal, D., Kumar, R., Banik, S., Sankaranarayanan, S. K. R. S., & Molinero, V. (2024). Medium-density amorphous ice unveils shear rate as a new dimension in water’s phase diagram. Proceedings of the National Academy of Sciences, 121(48). https://doi.org/10.1073/pnas.2414444121
Unlike crystalline ice, which shows a periodic atomic structure, amorphous ice lacks long-range order. It has traditionally been classified into two distinct forms: low-density amorphous (LDA) and high-density amorphous (HDA) ice. The discovery of MDA ice, first observed through ball milling techniques, suggested the existence of an intermediate phase. The new study led by postdoctoral researcher Ingrid De Almeida Ribeiro explores how shear forces influence the transition between these amorphous states as well as changes in temperature and pressure.
Ribeiro stated:
“Think about walking into a movie theatre. All the seats are lined up in perfect rows and columns. That’s like crystalline ice; atoms arranged in a structured, repeating pattern. Now, picture a music festival, people are just scattered everywhere; some packed closely together, others with more space between them, no clear arrangement. That’s like amorphous ice.”
She went onto say:
“Now, picture a liquid where atoms move freely. If you were to freeze that disordered structure without allowing the atoms to rearrange into a crystal, you’d get a glass. It’s like a frozen snapshot of a liquid.”
Ball milling, which is a process involving the agitation of ice samples with stainless steel balls, has previously been used to bring about structural changes in materials. In this study, researchers simulated shear deformation using high-performance computing, applying shear rates up to 100 million times per second to observe how molecular structures responded under stress.
This was done by using a sample of ice along with stainless steel balls which are contained within a sealed jar and kept at a temperature of 77 Kelvin. These simulations showed that shear induces a restructuring of water molecules, forming intermediate-density amorphous structures distinct from LDA and HDA.
One of the study’s key findings is the role of shear as a fundamental factor in water’s phase diagram. Traditional thermodynamic pathways, such as temperature and pressure changes, dictate the formation of ice structures. However, the introduction of shear provides a new dimension to phase transitions in amorphous ice. Under shear, ice grains reorganised into new amorphous forms, bridging the density gap between LDA and HDA.
Modern ice production through conventional cooling methods does not yield MDA ice but this new process enables scientists to achieve it. The shear-driven process of ball milling enables scientists to discover brand-new amorphous structural forms that were before inaccessible. Researchers can shed light on glassy material behaviours by controlling these states when studying water-based materials and additional materials like silicon silica and carbon-based structures.
Scientists focus beyond atmospheric research. This is due to amorphous ice representing the most common solid water structure throughout the universe. The frozen surface of Jupiter’s Europa along with Saturn’s Enceladus creates suitable conditions to produce amorphous ice because these bodies possess very cold temperatures and extremely thin atmospheres. Research data indicates that extraterrestrial ice compositions could be shaped by shearing effects which scientists previously overlooked.
Professor Valeria Molinero led this study in collaboration with scientists from Argonne National Laboratory participated in demonstrating the essential role of computational modelling for modern materials science research. Shear recognition as a fundamental variable regulating phase transformations creates new prospects to modify amorphous solids both in terms of structure and properties.
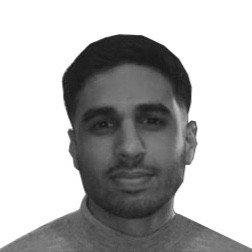
Hassan graduated with a Master’s degree in Chemical Engineering from the University of Chester (UK). He currently works as a design engineering consultant for one of the largest engineering firms in the world along with being an associate member of the Institute of Chemical Engineers (IChemE).