In a collaborative research effort at the research led by TU Delft, in collaboration with the University of Valencia and the National University of Singapore, Dr. Makars ŠIŠKINS have turned atomically thin FePS₃ membranes (nanodrums) into ultrasensitive probes of magnetic phase transitions in two-dimensional materials.
By tracking shifts in resonance frequency, quality factor and nonlinear damping as the membrane crosses the Néel temperature, they unveil the intimate coupling between spin order and mechanics without the need for large magnetic fields or bulky instrumentation. Their research, published in Nature Communications and covered in our previous news feature, shows how strain and high-amplitude motion can be used to tune and read out antiferromagnetic ordering via purely mechanical means. The research can be found here:
Šiškins, M., Keşkekler, A., Houmes, M. J. A., Mañas-Valero, S., Koperski, M., Coronado, E., Blanter, Y. M., van der Zant, H. S. J., Steeneken, P. G., & Alijani, F. (2025). Nonlinear dynamics and magneto-elasticity of nanodrums near the phase transition. Nature Communications, 16(1), 2177. https://doi.org/10.1038/s41467-025-57317-4
The latest research produced by the team can be found below:
Šiškins, M., Keşkekler, A., Houmes, M.J.A. et al. Nonlinear dynamics and magneto-elasticity of nanodrums near the phase transition. Nat Commun 16, 2177 (2025). https://doi.org/10.1038/s41467-025-57317-4
Houmes, M.J.A., Baglioni, G., Šiškins, M. et al. Magnetic order in 2D antiferromagnets revealed by spontaneous anisotropic magnetostriction. Nat Commun 14, 8503 (2023). https://doi.org/10.1038/s41467-023-44180-4
Šiškins, M., Lee, M., Mañas-Valero, S. et al. Magnetic and electronic phase transitions probed by nanomechanical resonators. Nat Commun 11, 2698 (2020). https://doi.org/10.1038/s41467-020-16430-2
The following interview is presented unedited to preserve Dr. ŠIŠKINS’s original insights into nanodrum design, dynamic spin-lattice coupling and the exciting future of mechanically reconfigurable magnetic devices.
Could you explain how dynamics of nanodrums function as a tool for studying magnetic phase transitions in 2D materials, and what advantages they offer over conventional characterisation techniques?
Nanodrums, which are essentially ultra-thin membranes suspended like tiny drums, are incredibly sensitive to changes in material properties these are made of. In this study, we used them to explore how the mechanical behavior of a 2D magnetic material changes when it goes through a phase transition. As the membrane’s material shifts from a disordered to an ordered magnetic state, the vibrations of the nanodrum respond in unique ways — especially in how energy is lost or “dissipated.” Unlike traditional techniques (e.g. magnetometry) which usually measure magnetisation directly, our nanodrum-based method is contactless, operates at small sample scales, and is inherently sensitive to internal dissipative mechanisms that are typically hard to access.
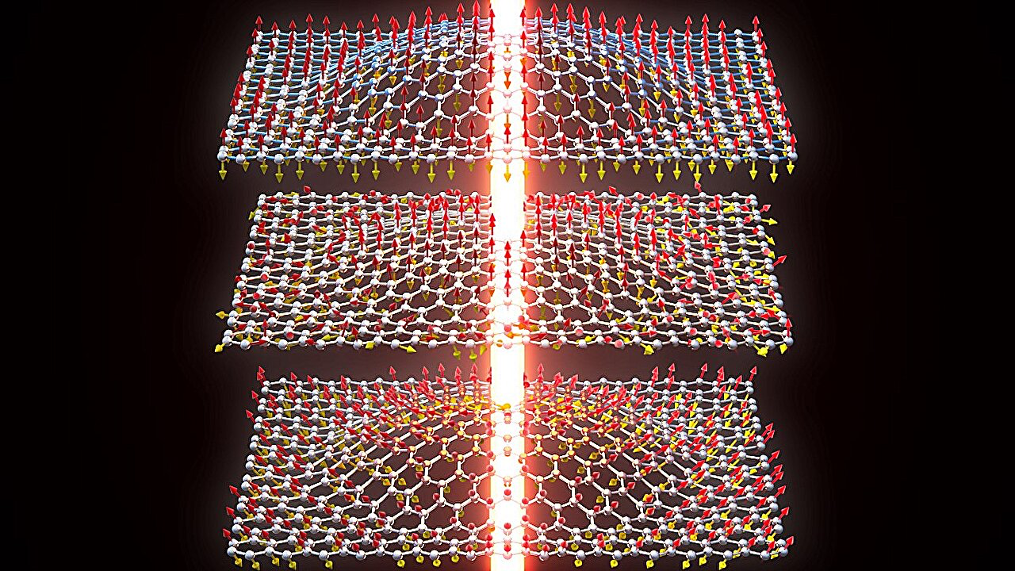
Essentially, what we’ve shown is that nanodrums can act like precision sensors, capturing fine details about how magnetism and mechanics are intertwined — all without needing large magnetic fields or bulky equipment.
What specific 2D materials were investigated in this study, and what unique magnetic behaviours were observed under different external conditions such as strain and temperature variations?
We focused on a layered magnetic material called FePS₃. It’s only a few atomic layers thick, but it goes through a clear magnetic transformation when cooled – switching from a paramagnetic to an antiferromagnetic state. What we previously found is that just as this transition occurs, the membrane’s linear low-amplitude vibrations begin to behave in unexpected ways, producing anomalies in temperature-dependent resonance frequency and quality factor. Furthermore, we discovered that when we apply larger excitation force, driving the membrane into nonlinear high-amplitude regime, there’s a sharp increase in energy dissipation and the stiffness of the nanodrum changes noticeably. It shows just how sensitive this system is to both internal and external conditions – how magnetism can have a real, measurable effect on how the material moves.
How does the confinement of these materials in nanodrum structures affect their magnetic ordering, and what insights does this provide into their phase transition dynamics?
The nanodrum device arrangement does more than just hold the material — it actually shapes its behavior. Because the membrane is free-standing, it is free to move – vibrate, flex and stretch in contrast to that on a solid surface. As the device is cooled, this results in the accumulation of tensile strain, which directly influences the membrane’s mechanical resonance frequency. Since it is sensitive to magnetic interactions in FePS₃, this setup offers a precise way to probe how magnetic ordering modulates strain, particularly near the Néel temperature. Now, during each oscillation cycle, the membrane also strains itself dynamically, effectively reorganizing magnetic configurations slightly under time-dependent stress. Importantly, this dynamic interaction modifies its energy dissipation and stiffness — signatures we can also track via resonance measurements.
What role do external stimuli, such as mechanical strain or magnetic fields, play in tuning the nonlinear dynamics and magnetic properties, and how could this be exploited for future applications?
External stimuli like strain can tune the mechanical tension in the membrane, which in turn modulates the antiferromagnetic order parameter via spin-lattice coupling. When driven to nonlinear regime of motion, the membrane reaches such high amplitudes that it introduces dynamic strain which affects the magnetic order within a period of vibration. However, the responce of such magnetic system to changes in strain – or vise versa, relaxation of strain in responce to the change in magnetic order – takes time. We found that this delay led to dramatic changes in nonlinear damping, indicating a strong interaction between mechanical and magnetic behaviour. This way we can introduce another tuning knob for these systems – additionally to an external magnetic field – which now can modulate the spin alignment dynamically. In future applications, this tunability could enable reconfigurable magnetic sensors or strain-controlled spintronic devices, where high-frequency logic or memory elements can be written and erased mechanically rather than electro-magnetically.
How do the findings of this research contribute to potential advancements in spintronics or quantum computing, particularly in the context of designing reconfigurable magnetic materials?
The strong magneto-mechanical coupling we revealed offers a mechanism for real-time high frequency control of magnetic phase transitions, potentially without applying magnetic fields – only by dynamic strain modulation. For spintronics, this suggests new paradigms for low-power, reconfigurable devices. In quantum systems, where coherence and environmental coupling are critical, understanding dissipative mechanisms like nonlinear damping becomes essential. We believe that in future our nanodrum approach could also be used to explore quantum-critical phenomena and quantum phase transitions – important steps toward hybrid quantum systems.
What were the key challenges in integrating nanodrum-based methodologies with existing experimental techniques, and how were they addressed in this study?
One major challenge was to combine the best of both worlds: achieving stable, high-Q mechanical resonators made from atomically thin 2D membranes, while at the same time integrating a material with very prominent spin-lattice coupling. Another challenge was the extreme sensitivity required for interferometric detection at cryogenic temperatures. This was achieved by carefully engineering the high-stability optical laser interferometry setup. Additionally, correlating the mechanical response with magnetic phase transitions required a cryogenic system with a precise thermal control and powerful magnet for fine sweeps around the Néel point.
Looking ahead, what are the next steps for your research team? Are there specific material systems or applications you are aiming to explore using nanodrums in the future?
We’re very excited to apply this approach to other magnetic 2D materials — including those with more exotic properties like frustrated magnetism or more complex magnetic behavior. Another exciting avenue is using time-resolved techniques to probe the dynamics of phase transitions far from equilibrium – to push into non-equilibrium dynamics, studying what happens when we rapidly drive these systems through phase transitions. On the device side, we are also looking into creating nanodrum arrays for magnetic sensing and coupling to spin waves, which eventually could result in transducers that bridge mechanical and spin-based platforms.
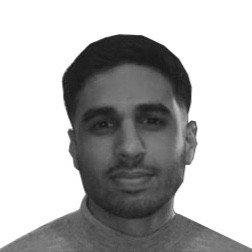
Hassan graduated with a Master’s degree in Chemical Engineering from the University of Chester (UK). He currently works as a design engineering consultant for one of the largest engineering firms in the world along with being an associate member of the Institute of Chemical Engineers (IChemE).