Researchers at the University of Vienna have unveiled the accordion-style rippling effect in graphene by developing an ultra-clean, air-free transfer system; reshaping our understanding of 2D material mechanics. In our earlier news report, we examined how this breakthrough challenges existing knowledge about vacancy-driven properties in graphene. Now, we sat down with Dr. Kotakoski (Vice Dean, Faculty of Physics; Head, Physics of Nanostructured Materials) and Wael Joudi to delve deeper into their experimental design, the surprises they encountered, and the broader implications for both fundamental science and future applications. You can find more information on the University of Vienna’s official press release. The research paper this interview pertains to can be found here:
Joudi, W., Windisch, R. S., Trentino, A., Propst, D., Madsen, J., Susi, T., Mangler, C., Mustonen, K., Libisch, F., & Kotakoski, J. (2025). Corrugation-Dominated Mechanical Softening of Defect-Engineered Graphene. Physical Review Letters, 134(16), 166102. https://doi.org/10.1103/PhysRevLett.134.166102
Follow some of the authors here:
- Wael Joudi | LinkedIn
- Dr. Jani Kotakoski | LinkedIn
- Rika Saskia Windisch | Linkedin
- Alberto Trentino | Linkedin
- Diana Propst
- Florian Libisch | Linkedin
The following interview is presented unedited to preserve their original responses and give you an unfiltered view of how they achieved full environmental control, integrated theory with practice, and engineered a multi-instrument, interference-free platform to be able to test accordion-style flexibility in other 2D crystals. The research was funded in whole or in part by the Austrian Science Fund (FWF).
How did your insights into 2D material behaviour drive the design of the ultra-clean, airless transfer system that revealed the accordion rippling effect in graphene?
We were surprised by some of the results reported in the literature, and wondered whether the discrepancies could be explained by uncertanties arising from uncontrolled experimental environment. Because of this, we developed the setup used here to reveal what happens when the experiment is carried out with full control.
What criteria did you use to determine which vacancy densities and configurations to introduce into the graphene lattice, and how did those choices influence the onset of corrugation?
There were already many reports on the effect of vacancies on the mechanical stiffness of graphene, some of which are contradictory as we point out. From these, the order of magnitude for the vacancy densities was clear to us. As for the vacancy type, we know already from our past published study that the atomic scale manipulation technique that we use creates mostly vacancies with two missing atoms. Therefore, it wasn’t so much an intentional choice for that type as it was a natural result of the technique.
Which observations about vacancy-induced bulging or wave formation surprised you most, and how have they reshaped your understanding of defect mechanics in graphene?
The vacancy-induced bulging in graphene is a phenomenon that has been revealed by computational studies already many years ago, so seeing indications of this in our atomic resolution images was not much of a surprise. The novelty here is the connection of these bulges caused by vacancies to the in-plane stiffness of the material. It surprised us how dominant their role was in the softening.
How has the discovery that surface contaminants suppress the rippling effect informed your lab’s protocols for handling and characterising other 2D materials?
The lab protocol and experimental procedure in our group has been developing for about a decade now. Since our focus is researching 2D materials at the atomic scale, isolating our materials from external foreign atoms as well as possible has always been our goal. This study shows an extreme case where neglecting this approach can have massive effects on the measured results and further confirms the validity and necessity of our lab protocol and experimental setup.
In coordinating with theoretical teams at your institution, how did you integrate simulation feedback into your experimental design, and what partnerships proved most critical?
The theoretical insight from simulations further confirming our experimental findings and at that time hypothetical model was provided by physicists of the Technical University of Vienna. This was a valuable collaboration since at that time the model we had developed was not distinguishing between vacancy types. The results of our collaborators showed that only vacancies with at least two missing atoms contribute to the corrugation. Implementing this insight improved the accuracy of our model.
What were the biggest technical hurdles in maintaining an interference-free environment across multiple instruments, and how did your group overcome them?
The challenges that came with that goal are three-fold. Firstly we had to work out intercompatibility across multiple different devices. These are produced and sold by individual companies, whereby each company has a different solution for sample holders and none of these are made with an interconnected vacuum system in mind. As a result, we had to come up with our own sample holders and transport infrastructure that was compatible with every instrument in our system. A good example of this is the custom sample stage of the Nion UltraSTEM 100, which was specially designed to be compatible with our sample holders. Secondly, the space requirements have to be met for both the large instruments and vacuum chambers as well as the vacuum tubes connecting them.
As of today the entire system spans over two floors. Thirdly, also the location of the laboratory is very important. For precise imaging and measurements at the atomic scale mechanical vibrations caused by nearby vehicles or strong electromagnetic field emerging from tram lines can lead to issues. Our lab is located in the old observatory of the University of Vienna located in a middle of a park, which fulfils even the most stringent requirements for disturbance-free measurements.
Looking ahead, which 2D materials or device architectures are you most excited to test for accordion-style flexibility, and what applications do you envision?
We believe that this effect is limited to graphene, due to the flexibility of carbon atoms to rearrange in response to local disorder. In follow-up experiments, we want to test this hypothesis by repeating the experiments with other 2D materials, such as hexagonal boron nitride.
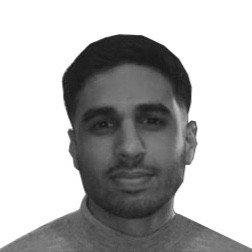
Hassan graduated with a Master’s degree in Chemical Engineering from the University of Chester (UK). He currently works as a design engineering consultant for one of the largest engineering firms in the world along with being an associate member of the Institute of Chemical Engineers (IChemE).